
For example, long-lived tree species of stable plant communities are usually predominantly outcrossing whereas weedy colonists that occupy ephemeral environments are more commonly highly selfing. Ecological factors play a key role in driving the evolution of reproductive traits and in the maintenance of different mating systems. This variation implies frequent evolutionary transitions between mating systems, which are usually associated with changes to floral characters, pollination systems, and sex allocation in concert with life-history evolution. Reproductive versatility is therefore a hallmark of angiosperm evolution and this is manifested by considerable inter- and intra-specific variation in mating systems. The diversification of many angiosperm families (e.g., the orchid and phlox families) has been attributed to adaptive radiation of pollination and mating systems, often accompanying changes in the ecology of populations. Crowson, in Encyclopedia of Evolutionary Biology, 2016 Evolution of Mating SystemsĪmong life-history traits, reproductive characters that influence mating are of profound adaptive significance because they govern the character of genetic transmission between generations, the fitness of offspring and the amounts and distribution of genetic diversity in populations.
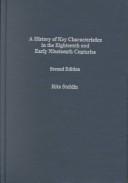
The dimensional analysis thus provides the theoretical background for constructing dimensionless numbers (e.g., ratio between age at first reproduction and lifespan) that are expected to be size-independent (see Charnov, 1993). Indeed, physical constraints lead us to expect isometric relationships among traits sharing the same dimension. For instance, the dimensional analysis implies an allometric exponent to one-third (not 1) when regressing antler size against body mass (on a log–log scale) to assess the type of allometry (i.e., positive, negative, or isometry). Such an heterogeneity in dimension has obvious practical consequences. For instance, gestation and incubation periods, weaning or hatchling periods, age at first reproduction, and lifespan are all measured in units of times (i.e., days, weeks, months, or years), antler size of deer is measured in units of length (centimeters or meters), and body mass has a dimension of volume measured in units of mass. Lebreton, in Encyclopedia of Evolutionary Biology, 2016Īll life history traits can be related to a fundamental dimension, or derived units. If it has an aggregated, patchy, spatial distribution (e.g., metapopulation structure) the degree of connectedness between the parts of the population and the degree to which they fluctuate independently can influence the extent of the variation of the whole population. Physiological and/or morphological changes (usually triggered by habitat characteristics) can lead to dramatic increases in population growth, for example, the shift from sexual to asexual reproduction when conditions are good.Īn organism's mobility (or its ability to disperse propagules) can also influence its temporal variability. Some species have reproductive strategies that amplify fluctuations in density. Whether such a definition is useful will depend on the question under investigation.

Arguably, the variation is the result of a particular definition of density. Life history characteristics, such as synchronized generations or life stages (perhaps dormant) that are not available for sampling (e.g., the seed bank or the nymphs of the 21-year cicada), can lead to apparently major changes in density. McArdle, in Encyclopedia of Biodiversity (Second Edition), 2013 Life History Characteristics Perhaps even more important, we need to know how the species respond to human activity (e.g., harvest, disturbance, and dispersal) because changes in human activity probably will be more important than climate in determining future vegetation composition over the coming decades.īrian H. However, to predict community change we need to know much more about life-history traits that determine dispersal and establishment.
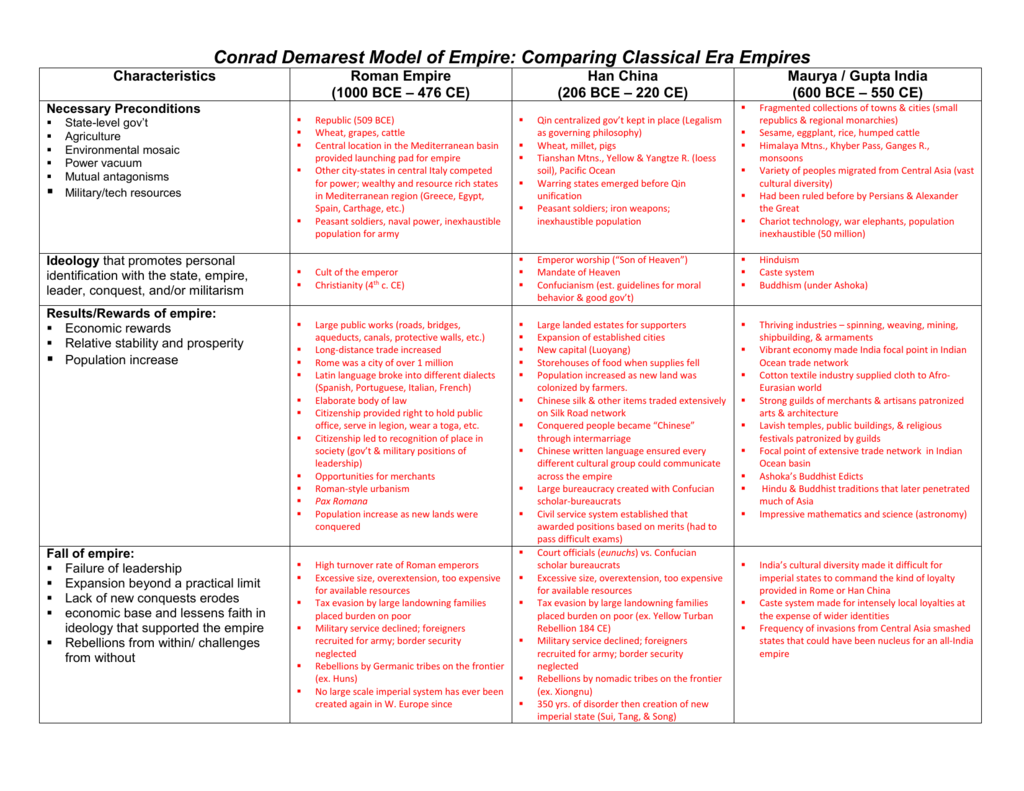
Any growth-form classification that links ecophysiological and ecosystem characteristics should, therefore, incorporate these traits (e.g., Table 16.1). RGR, tissue chemistry, phenology, and storage are other traits of major importance. In summary, plant size is the trait that most frequently influences ecosystem processes. In early primary succession, presence or absence of nitrogen fixers also strongly influences the rate and pathway of succession ( Vitousek and Walker, 1989). Life-history traits such as dispersal ability, reproductive potential, and degree of incorporation into the seed bank are probably the most important traits governing community change, both through succession ( Walker and Chapin, 1987) and during long-term vegetation change ( Davis, 1981). Stuart Chapin III, in Scaling Physiological Processes, 1993 G Community Change
